Overview
Particles therapy
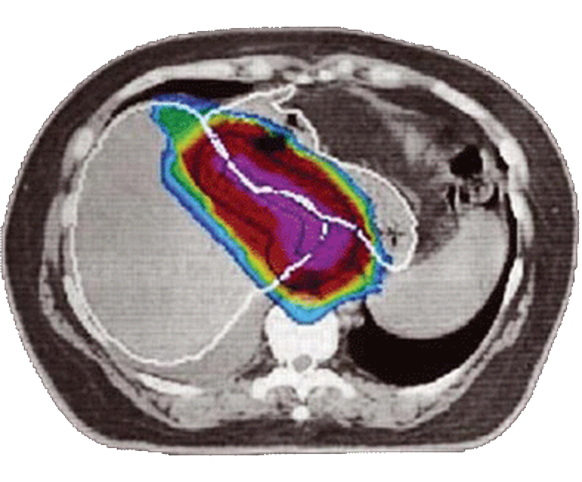
In recent years, the use of energetic hadrons for cancer radiation treatment has grown in importance among all major industrialized nations. The most recent studies report how radiation treatment using protons (1H) and carbon ions (12C) leads to improvements in treatment of many cancer forms [1]. Modern superconducting cyclotrons are compact machines, which make possible the acceleration of protons up to 250 MeV and 12C ions up to about 500 MeV, enabling the practical realization of hadron therapy facilities worldwide [2].
By using hadrons (protons and heavier ions) it is in fact possible to pinpoint the particles energy delivery into the tumor volume with millimetric precision, sparing nearby tissues from damage. Hadron therapy is therefore in the process of joining the already established X-ray therapy as an effective radiation therapy to treat cancer disease. However, the exceptional aiming precision theoretically achievable with particles therapy relies upon the precise knowledge of the tissues density along the particles path.
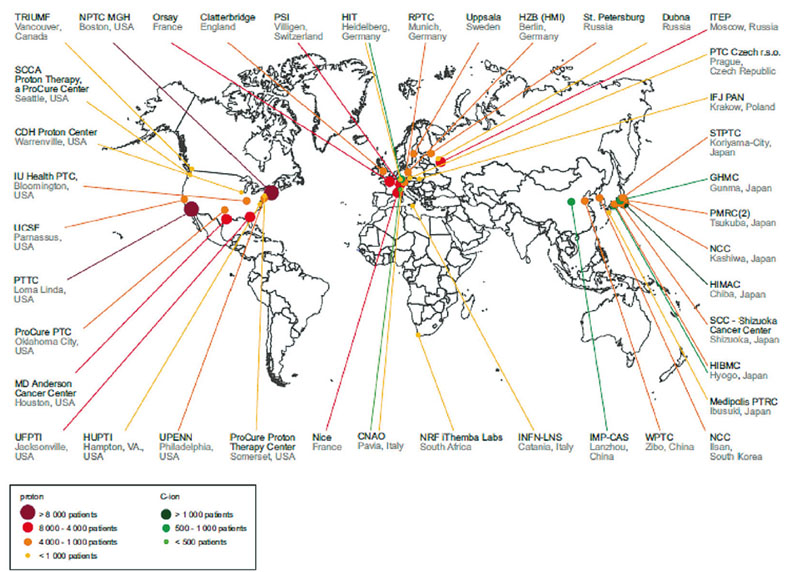
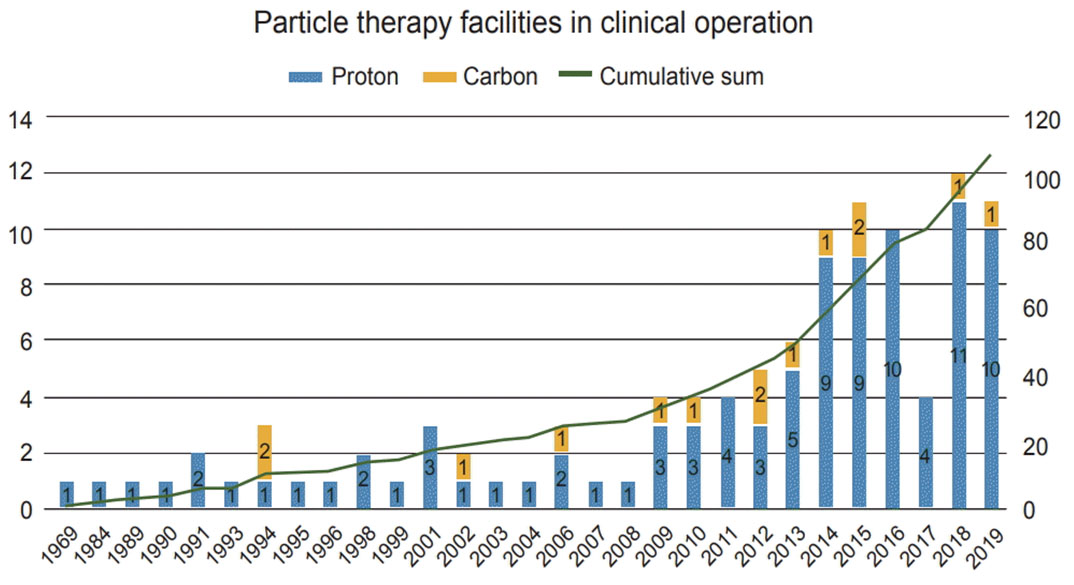
The lack of the precise 3D tissue density maps necessary to accurately tune the beam energy actually represents the major factor limiting the precision in tumours hadron therapy treatment [1]. Present facilities must in fact rely on X-rays CT data to plan the dose delivery, which leads to aiming errors much larger than the intrinsic precision proton therapy could achieve.
Proton CT
Nevertheless, CT imaging can be realized with particles different from photons: by using protons of energies between 200 and 300 MeV, it is in fact possible to obtain 3D images of the body with unprecedented tissue density resolution, and delivering a much lesser radiation dose to the patient. Hence, many groups are investigating the way to realize a proton CT (pCT) [4]. Present state-of-the-art pCT prototypes made excellent advancements in the field, and most advanced systems [5] greatly improved in term of speed and practical operability over the past generation prototypes. An effective pCT system will also provide a 3D imaging tool delivering much less total dose (< 2 mGy) respect to a standard X-ray based CTs [6].
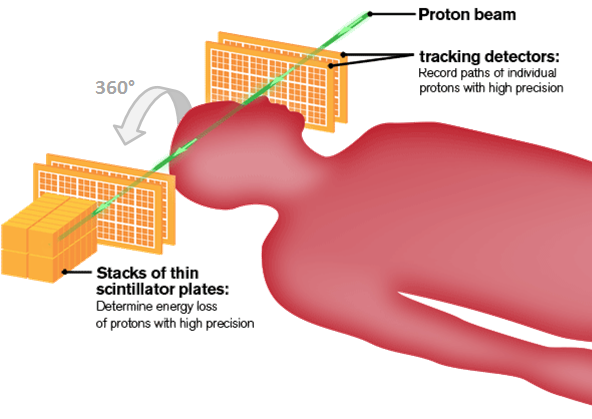
iMPACT
Parameter | Spec | Notes |
---|---|---|
Target volume | 15×15×15 cm3 | Human head size |
Beam raster area | 20×20 cm2 | Acceptance at the tracker |
Tracking speed | 100 MHz | Whole area, 10 MHz |
10 MHz | Pencil beam | |
Energy range | 100 – 250 MeV | Protons |
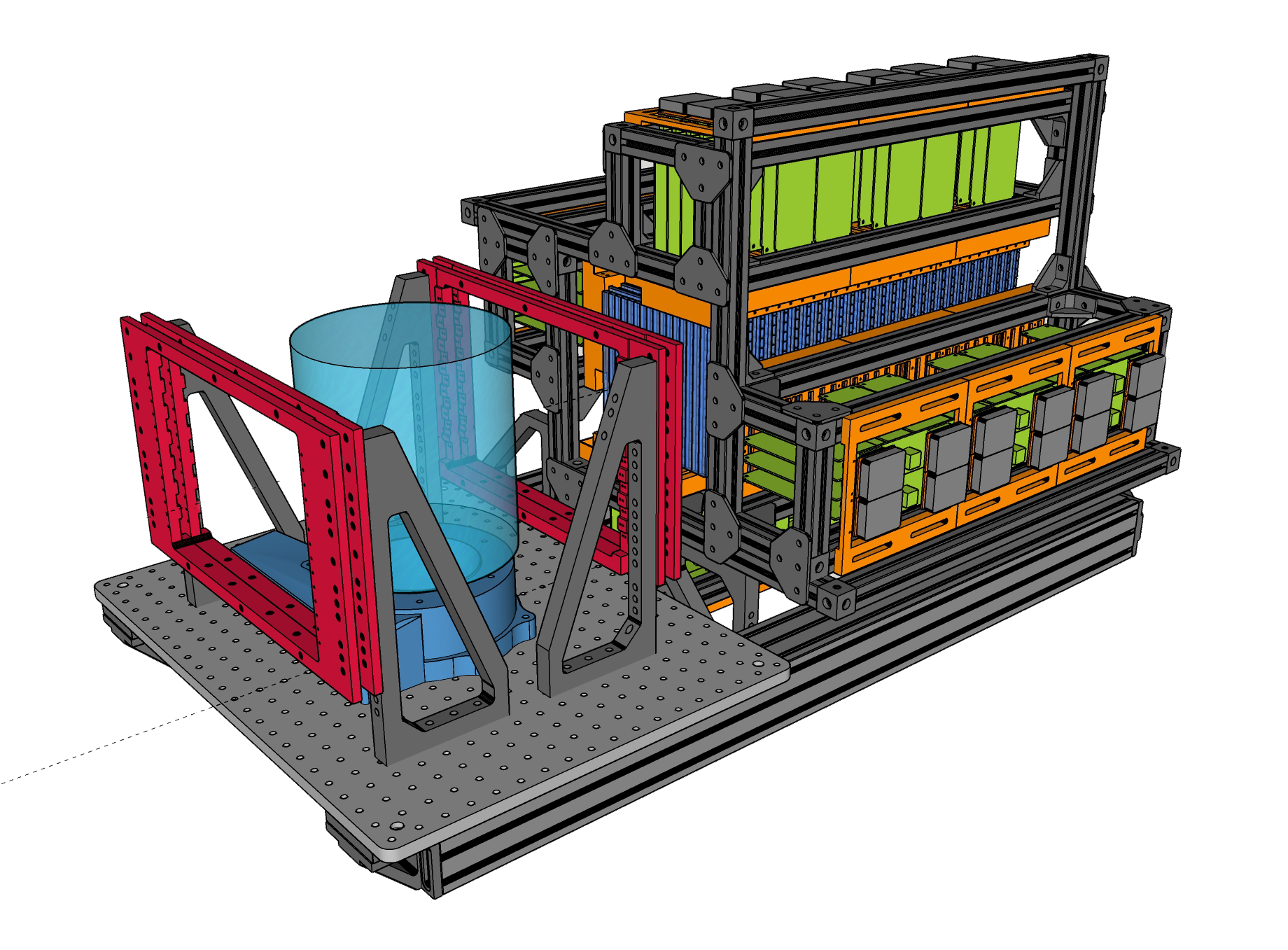
The iMPACT project (innovative Medical Protons Achromatic Calorimeter & Tracker) aims at building a pCT scanner further improving the current state-of-theart. Like present solutions, the iMPACT proton scanner consists of a tracker and a calorimeter.
The tracker is realized with 4 layers of Monolithic Active Pixel Sensors (MAPS), and in the present configuration uses 20 50µm thin ALPIDE sensor of 3×1.5 cm2 area each [7] per layer. A current R&D is ongoing within the INFN ARCADIA ptoject to realize a more advanced, larger sensor specifically tailored for the pCT application [8].
The calorimeter is instead a novel design, based on the idea of the range calorimeter, which measures the particle (dE/dx) to determine the occurrence of its maximum in space, hence deriving the particle residual energy after it passed through the target.
Latest results
Thesis works
Available topics
- 3D tracking and image reconstruction plus simulations
- Data Acquisition via FPGA and front-end electronics
- Apparatus development: large area sensor interface for ALPIDE chips
- Large dataset management
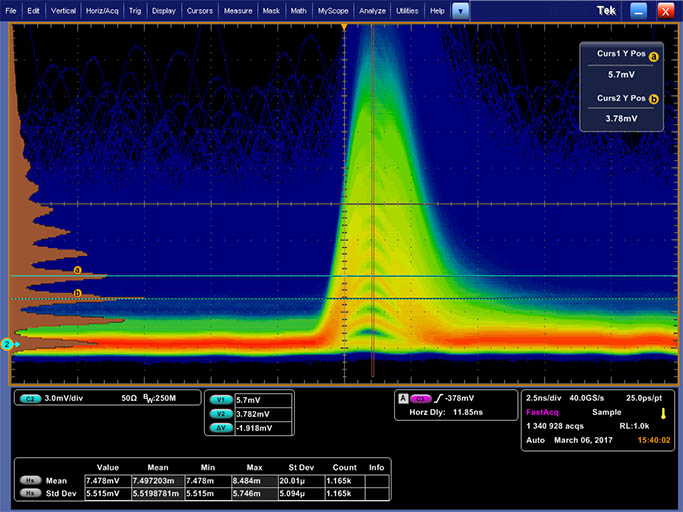
Acquired skills
- Programmatically reconstruct 3D imaged from the tracking dataset. Possibility to try GPU resources for Master and PhD students.
- Programming FPGAs and/or microcontroller, as well as advanced embedded systems (PXI).
- Organizing irradiation campaigns both with cosmic rays and at accelerators facilities.
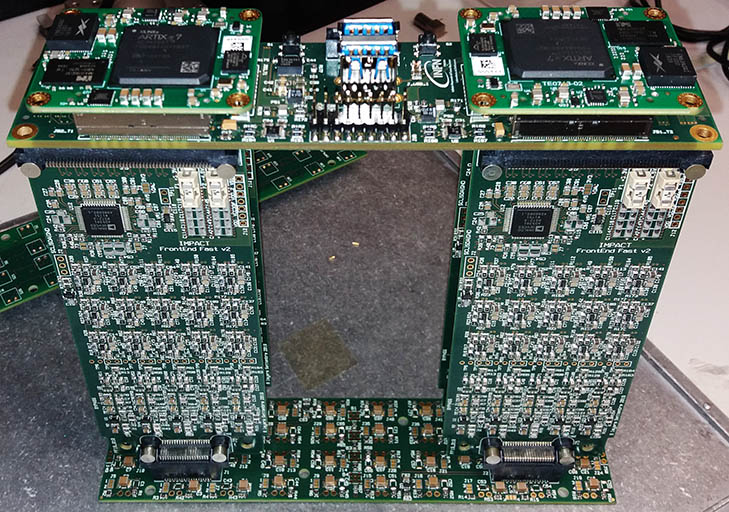
Funding
References
- Proton vs carbon ion beams in the definitive radiation treatment of cancer patients” – DOI: 10.1016/j.radonc.2010.01.015
- A review of ion sources for medical accelerators – 10.1063/1.3671744
- Toward Proton Computed Tomography – DOI: 10.1109/TNS.2003.823044
- Advanced proton imaging in computed tomography” – DOI: 10.1093/rpd/ncv197 210
- PRaVDA: The first solid-state system for proton computed tomography – DOI: 10.1016/j.ejmp.2018.10.020
- Review of medical radiography and tomography with proton beams – DOI: 10.1088/1361-6633/aa8b1d
- ALPIDE, the Monolithic Active Pixel Sensor for the ALICE ITS upgrade – DOI: 10.1016/j.nima.2015.09.057
- Sensor design optimization of innovative low-power, large area MAPS for HEP and applied science – arXiv:2011.09723