Research
Our Tools
Our International Collaborations

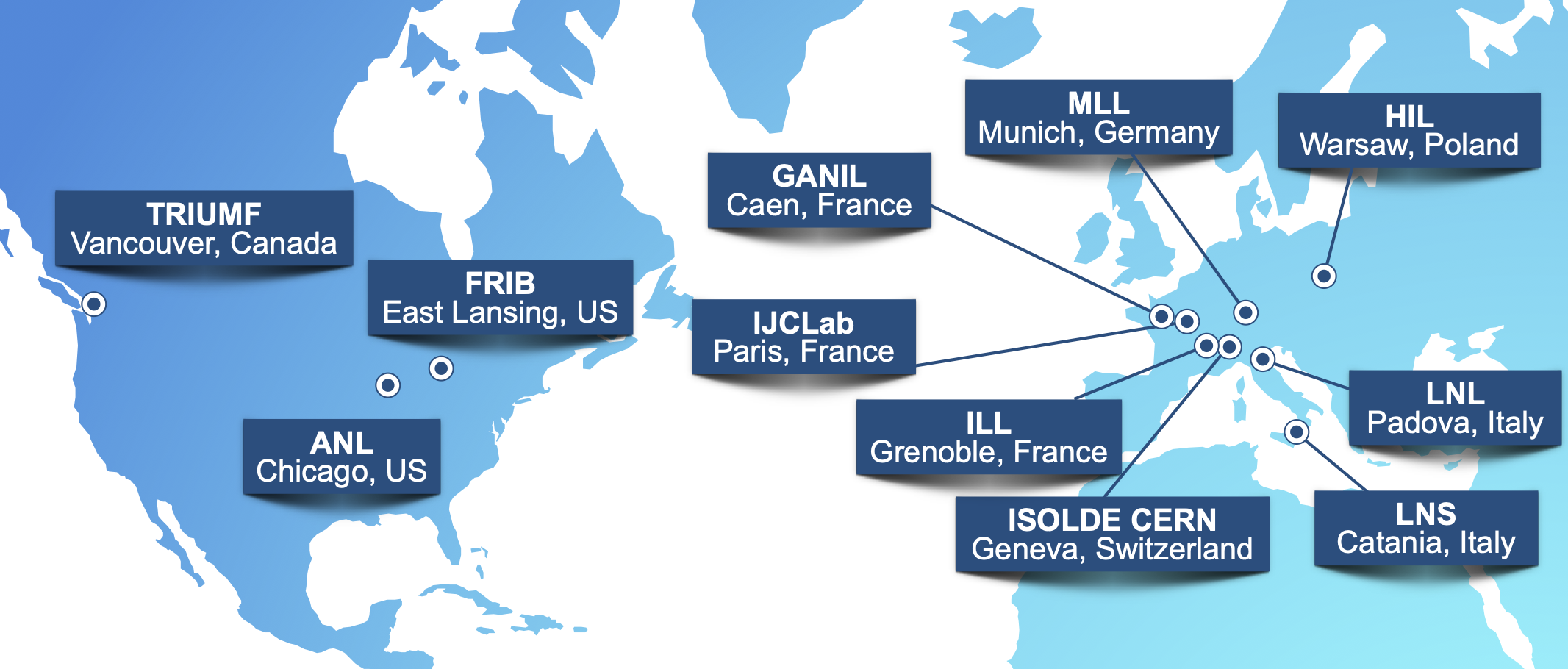
Our Labs
Active Projects
ARDE - Neural Network Algorithms for Discrimination of Electrons and Gamma-Rays
Principal investigator: Naomi Marchini
The ARDE project's goal is to conduct a comprehensive investigation into methods for distinguishing between electrons and gamma rays of nuclear origin in semiconductor detectors. The identification and measurement of these electrons, especially their energy determination, hold significant importance in both fundamental and applied physics research. Such studies encompass endeavours like exploring electric monopole transitions between nuclear states, which shed light on quantum phenomena like the coexistence of nuclear shape and nucleosynthesis of elements in stars, or detecting beta particles in radionuclides used for medical applications. One of the primary challenges in these measurements is the background contribution caused by gamma rays emitted during the de-excitation of the nuclear states under examination or due to natural radiation. ARDE aims to investigate techniques to substantially reduce this background in semiconductor electron detectors, mainly Si(Li) detectors, by leveraging signal shape analysis. These techniques will be pioneering the integration of "machine learning" methodologies for gamma-ray and electron discrimination, enabling the simultaneous utilisation of all the information contained in the collected signals. A successful outcome of this project will establish the foundation for similar advancements in the medical field and rare event physics.
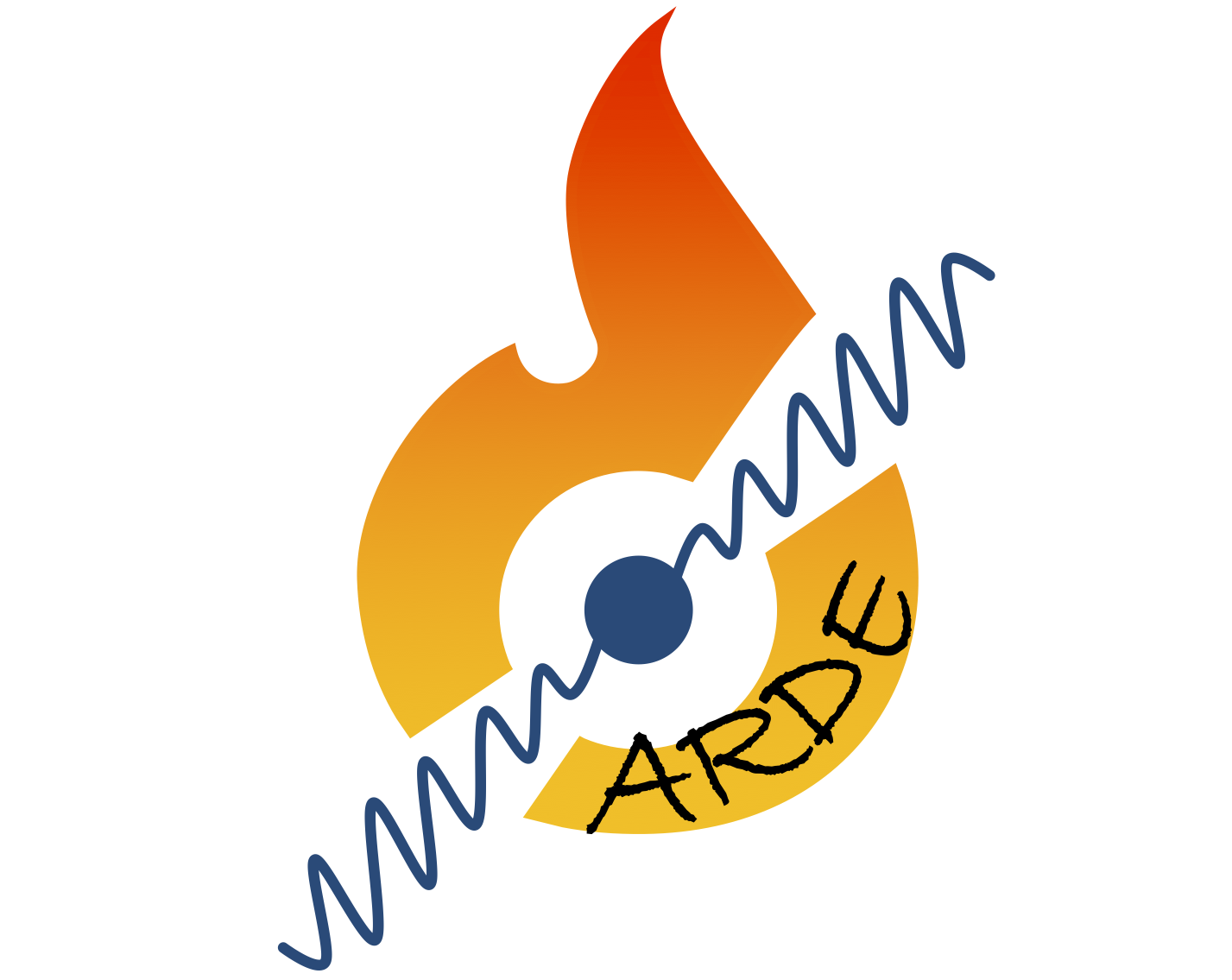
BeGam - Detector for Discrimination of Electrons and Gamma-Rays for Radiopharmaceuticals
Group principal investigator: Adriana Nannini
The BEGAM project addresses the isotopic purity of radiopharmaceuticals used in medical diagnostics from tomographic and planar imaging techniques. These techniques give the spatial distribution of a molecular probe labelled with a radionuclide and injected into the patient's body. After the injection, the outcoming radioactivity is registered by specific energy and space-sensitive detectors displaced outside the patient's body; for this reason, the use of radionuclides having a gamma emission associated with their beta decay is in order. The aim of BEGAM is the design and implementation of a portable and versatile setup, allowing for the detection of undesired pure beta emitter contaminants (which decay from ground state to ground state) inside the radiopharmaceutical. Such contaminants increase the dose absorbed by the patient, worsen the quality of the obtained image, and cannot be easily identified by employing commonly used dose calibrators.
The project is a collaboration with the Careggi Hospital in Florence, where the radionuclide will be produced at the GE MiniTrace 9.6 MeV cyclotron.
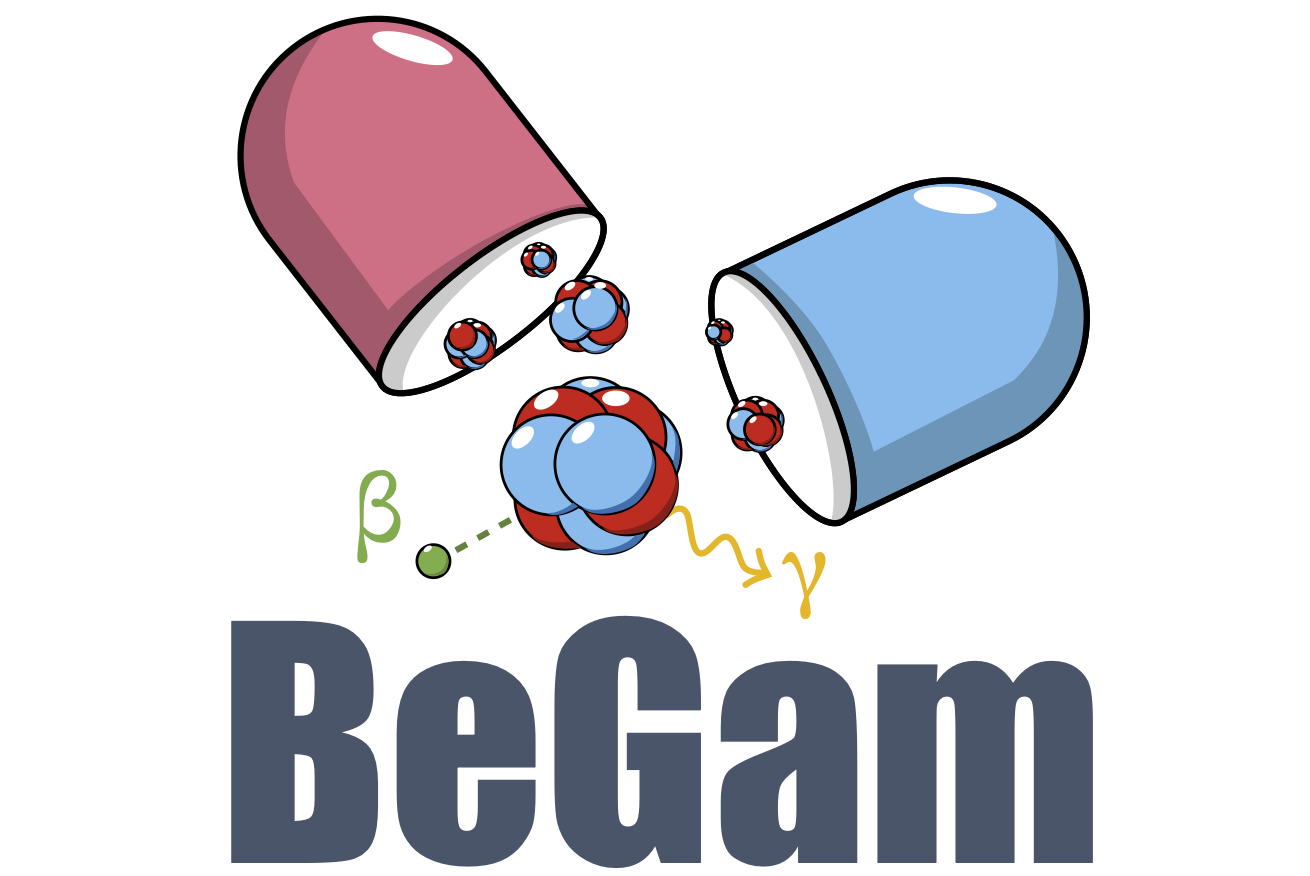
Probing Nuclear Vibrations: Revisiting a Fundamental Paradigm
Nuclear vibrations are one of the fundamental modes of excitation in atomic nuclei and have played a central role in the theoretical understanding of nuclear structure since the early models of Bohr and Mottelson. These collective oscillations of the nuclear surface have long been considered a universal feature of the low-energy spectrum of atomic nuclei, deeply connected to their shape and symmetry properties. However, in recent years, the validity and ubiquity of vibrational excitations have been questioned by new experimental evidence and refined theoretical models, suggesting that vibrational modes may not be as common or as harmonic as traditionally assumed.
Our research group is actively involved in this line of investigation, aiming to clarify the true nature of nuclear vibrations and their role in shaping the structure of atomic nuclei. To this end, we carry out precision experiments at leading international facilities, including TRIUMF in Canada, INFN-LNL in Italy, and Argonne National Laboratory in the United States. These studies are particularly focused on transitional nuclei, where the presence or absence of vibrational modes can signal changes in nuclear shape and symmetry. Ultimately, understanding the vibrational behaviour of nuclei is key to addressing a fundamental question in nuclear physics: are nuclei spherical, deformed, or something in between?
Contacts: Adriana Nannini (adriana.nannini@fi.infn.it)
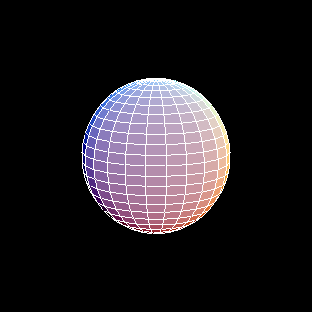
FAZIA (Forward A and Z ion Identification Array)
The project aims to conduct experiments utilizing a charged particle detector characterized by a wide solid angle coverage, excellent granularity, and high temporal resolution. FAZIA is specifically designed to maximize the isotopic separation of detected ions, employing both stable and radioactive ion beams. This capability facilitates the exploration of the thermodynamics and dynamics of exotic nuclei, delving into the isospin degrees of freedom (linked to the neutron-to-proton ratio) of nuclear matter. Additionally, the project sheds light on their role in the nuclear equation of state under high temperatures and nuclear densities, far from saturation.
Within the Fermi energy range (20-50 MeV/nucleon), a rich phenomenology is observed, ranging from binary reactions in peripheral collisions to multifragmentation events in central collisions. The reaction mechanism is influenced by both mean-field effects and nucleon-nucleon collisions. The theoretical framework predominantly relies on transport models, which have achieved considerable success, yet several crucial aspects still elude a complete modelling description.
The FAZIA project thus serves as a critical platform for experimental and theoretical investigations, pushing the boundaries of our understanding of nuclear matter and providing valuable insights into the complex dynamics of exotic nuclei.
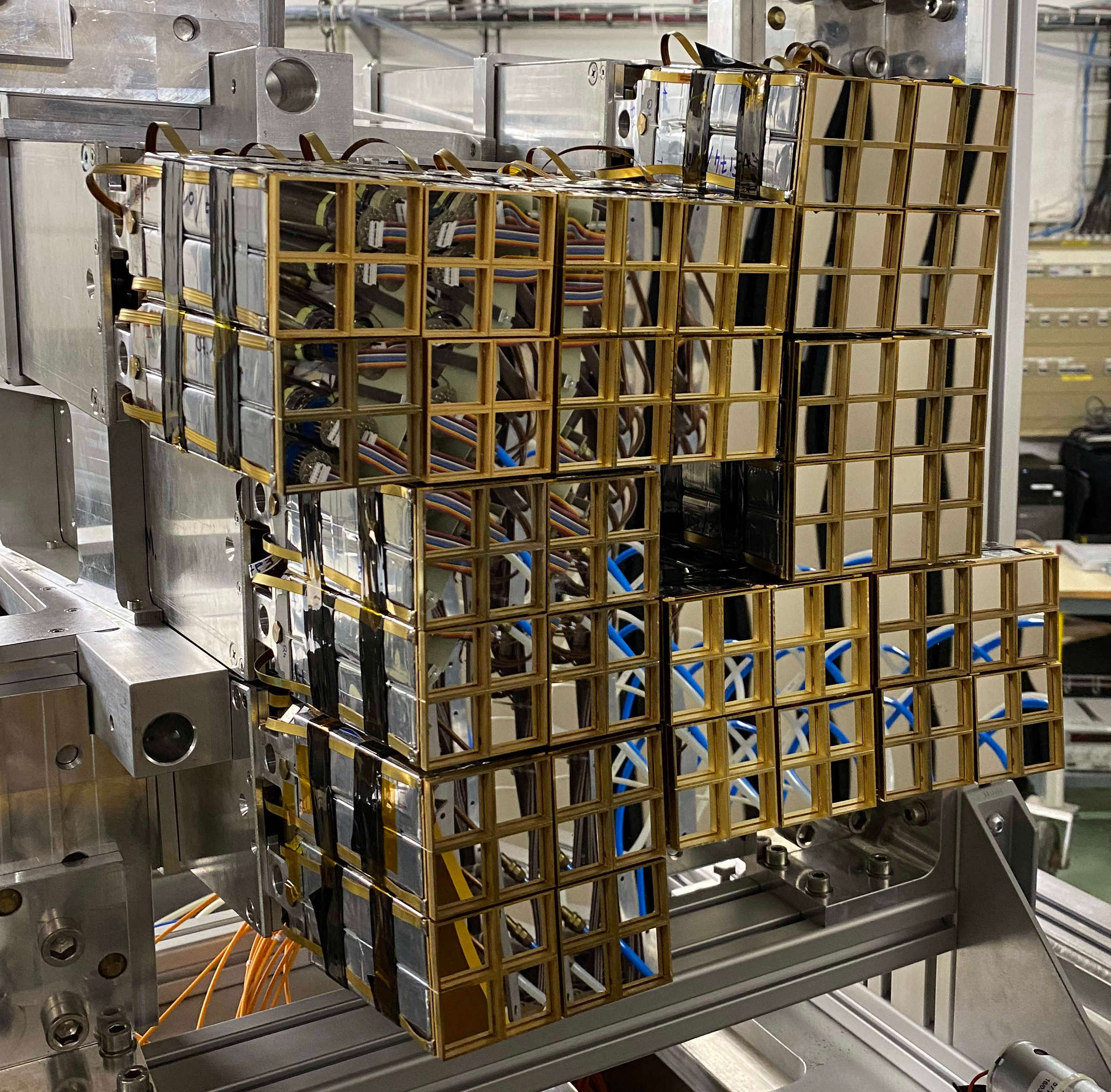
Shape and Structure of 130Te and 130Xe Relevant to Neutrinoless Double-Beta Decay
Contact: Marco Rocchini (marco.rocchini@fi.infn.it)
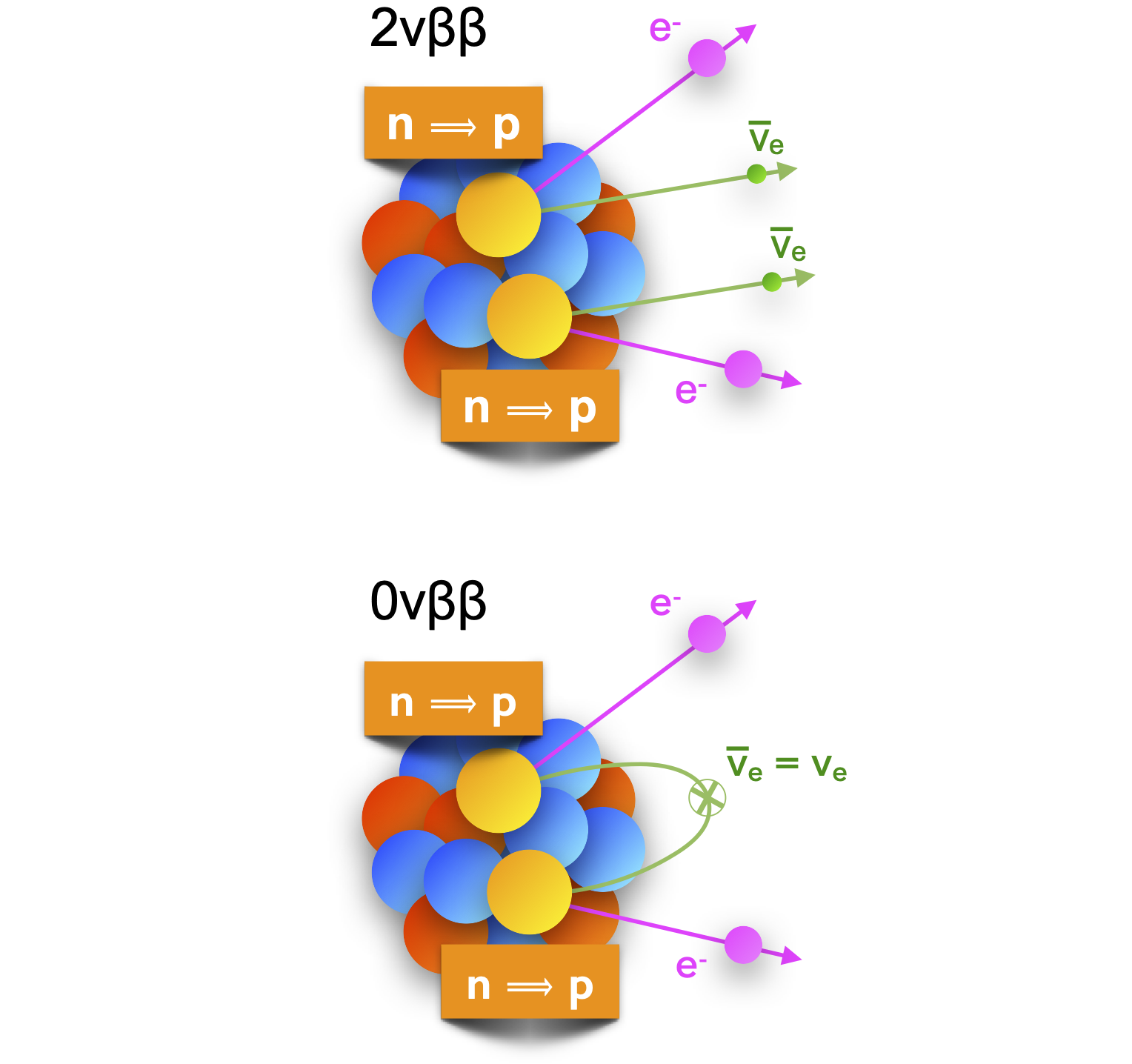
Development of New Semiconductor Devices for Nuclear Physics Studies with Radioactive Beams at SPES
The first RIBs from SPES are expected for 2024-2025. SPIDER and SLICES will be among the first instruments to be used in the experimental campaigns.
Contact: Adriana Nannini (adriana.nannini@fi.infn.it)
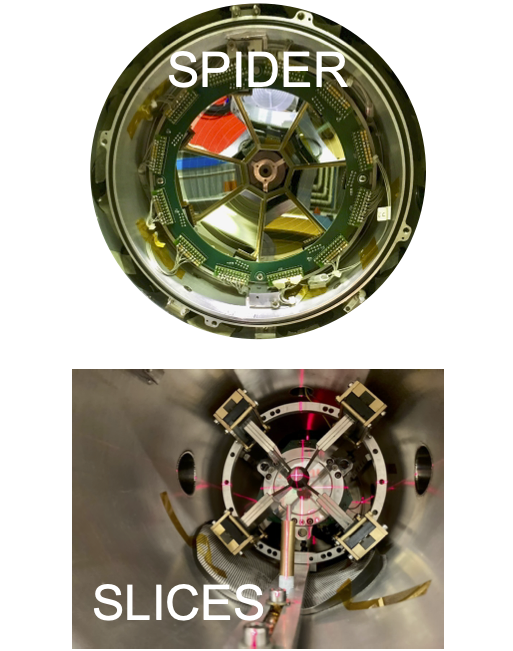
Atomic Nuclei Clustering
Diving into the heart of this mystery, researchers investigate diverse manifestations of clustering, ranging from alpha-like configurations in lighter nuclei to intricate structures in heavier isotopes. Advanced detectors and innovative methodologies are employed to discern the specific dynamics that give rise to these clustered formations.
Theoretical models, grounded in quantum mechanics and nuclear forces, play a pivotal role in interpreting experimental data, striving to capture the nuances of stability and energetics inherent in clustered configurations. The project not only refines existing models but also pioneers novel approaches to comprehensively understand the rich landscape of nuclear clustering.
Beyond contributing to fundamental nuclear structure knowledge, this research carries implications for astrophysical phenomena, such as nucleosynthesis in stellar environments. The exploration of nuclear clustering dynamics sheds light on the intricate interplay between nuclear forces, shell structure, and clustering phenomena, propelling advancements in our comprehension of the diverse and dynamic nature of atomic nuclei.
Contact: Alberto Camaiani (alberto.camaiani@fi.infn.it)
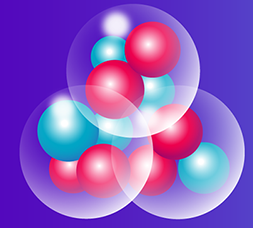
Type-I and Type-II Shell Evolution in the Zirconium Isotopes
Contacts: Naomi Marchini (naomi.marchini@fi.infn.it), Marco Rocchini (marco.rocchini@fi.infn.it)
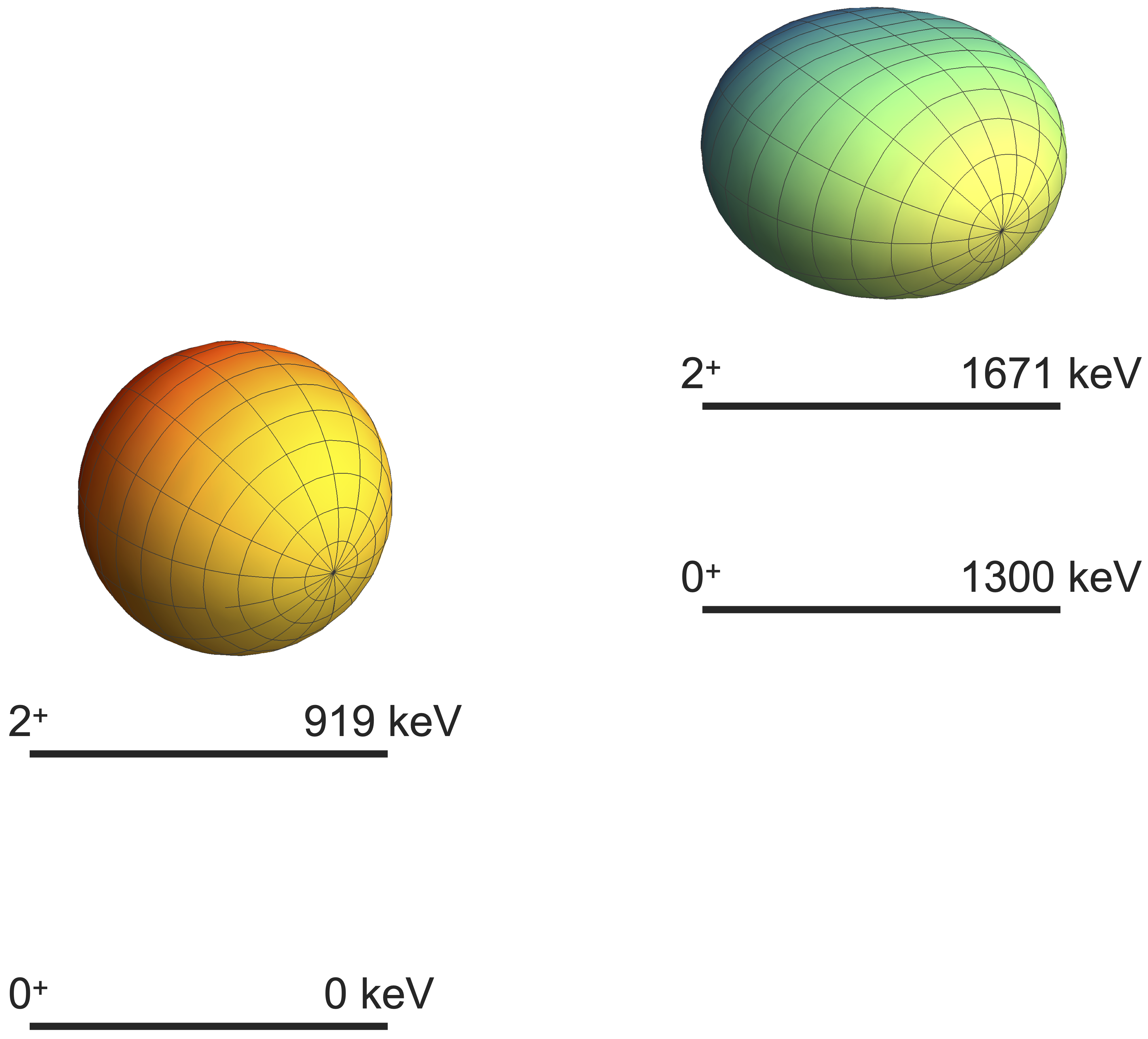